As part of its Prescription to Performance (P2P) initiative, the National Ready Mixed Concrete Association surveyed its members. They wanted to know which prescriptive requirements kept them from optimizing concrete. As we’ve discussed previously, excessive overdesign not only costs more, but also increases the concrete’s carbon footprint. Here are the five most onerous prescriptive requirements the NRMCA survey identified.
1. Water-cementitious materials ratio
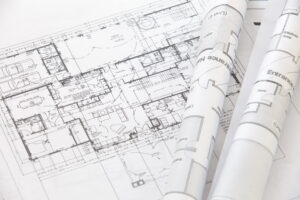
Sometimes limiting the water-cementitious materials ratio (w/cm) is necessary and appropriate. For example, concrete that will experience cycles of freezing and thawing in the presence of water and deicing salts should have a maximum w/cm of 0.45. Concrete in contact with sulfate soils also requires a low w/cm. ACI 318 outlines the concrete exposure classes that necessitate such provisions. But specifications often limit the w/cm even when ACI 318 doesn’t require it.
Why is that a problem? For one thing, it almost assuredly results in unnecessary overdesign. When a specification is too prescriptive, it restricts the contractor’s choice of means and methods to achieve the desired performance. Reducing the w/cm usually necessitates higher portland cement contents and/or admixture dosages, as well as tighter quality control. Better to let the contractor figure out a less costly way to do the job. That is, moving from prescription to performance gets us “more bang for the buck”.
Regardless of whether a low w/cm is necessary for durability, it will result in higher strength. If high strength isn’t necessary to the structural design, it will be less efficient than it could be. Wherever the specification calls for a low w/cm, the structural engineer should make use of the high strength. That may mean smaller columns, longer spans with fewer columns, or prestressing for a more sustainable structural design.
Low w/cm also results in higher stiffness and lower creep. These are beneficial in prestressed concrete, tall buildings, and other applications where you want to limit short- and long-term deflections. However, if you need to limit cracking, creep is your friend. And if you want a repair concrete that’s compatible with the substrate material, you may need to limit the stiffness.
2. Cementitious materials content
ACI 301 has a minimum cementitious materials content for concrete in interior floors, such as in a warehouse or industrial application. The purpose is to provide enough paste for a smooth, hard-troweled finish. Even then, a mockup slab would demonstrate how well the concrete finishes, as well as its tendency to curl or crack.
Before admixtures were in common use, a minimum cementitious materials content might have helped to ensure good concrete even if the contractor added water to make it more workable. But having more cementitious material than you need is not only wasteful; it may make the concrete more susceptible to shrinkage and cracking. In a floor slab, that means more curling as well.
3. Supplementary cementitious materials content
ACI 318 limits the amounts of supplementary cementitious materials for concrete subject to deicing salts to prevent surface scaling. Concrete for an F3 exposure class should have no more than 25% fly ash, 50% slag cement, or 10% silica fume. ACI 318 also limits combinations of these materials. However, many specifications impose these limits even on indoor concrete.
So what’s the problem? This prescriptive requirement may make it difficult to meet certain performance requirements. Resisting sulfate attack could easily require more than 50% slag cement. Mitigating expansions due to alkali-reactive aggregate might require more than 25% of some fly ashes, particularly Class C ash. So do you use the local Class C fly ash or import a Class F fly ash? If you can’t use more than 25%, importing Class F fly ash might be your only choice. A marine structure might use as much as 85% slag cement to resist sulfates and chlorides. A thermal control plan for mass concrete could require more than 50% slag cement to limit temperatures or control cracking.
Another reason to use supplementary cementitious materials is to reduce the carbon footprint of the concrete. Whether you’re conforming to CALGreen or you just want durable and sustainable concrete, you’ll want to use supplementary cementitious materials. Why impose arbitrary limits on them?
Some specifiers may limit supplementary cementitious materials for other reasons. For example, they may want to avoid delays in setting time or early-age strength gain. However, if those are their concerns they’d be better off directly specifying the setting time or 1-day strength—that is, the performance. Different fly ashes have dramatically different effects on these properties. Also, the contractor may prefer to obtain them with admixtures.
4. Aggregate characteristics
ASTM C33 is the standard for fine- and coarse aggregate. It contains broad limits on the particle-size grading for these aggregates, but says nothing about the grading of the combined coarse and fine aggregate. Optimizing aggregate grading can provide better workability, lower shrinkage, and less cracking. At Beton we use the aggregate suspension method in proportioning concrete mixtures.
However, these methods are best left to the contractor to decide. Some aggregate sources lack one or more size fractions. The supplier may have to waste some other fractions to meet a prescriptive requirement even if they could have achieved the desired performance. Or importing a suitable aggregate may be cost prohibitive. In addition, conformance to a specific aggregate grading does not guarantee performance. It’s better to specify the performance and let the contractor figure out how to get there. For example, if the concern is to control cracking, AASHTO T334 can test the cracking tendency directly. Or the specification can require a mockup to demonstrate the ease of pumping, consolidation, and finishing.
5. Supplementary cementitious materials characteristics
ASTM C618 sets the requirements for fly ash, as do ASTM C989 for slag cement and ASTM C1240 for silica fume. Concrete specifications incorporate these standards by reference. However, some specifications include further restrictions. For example, they may allow Class F but not Class C fly ash. Contractors are already contending with shortages of fly ash as more utilities close their coal-fired power plants. We ought to be using the fly ash we have so long as we can get the performance we want. Class F fly ash, which has less calcium than Class C fly ash, can usually control alkali-silica reaction at 15 to 25% of cementitious materials. Class C fly ash may require up to 35% to control the same aggregate. However, in the right proportions it can be every bit as effective.
A related requirement is a limit on the alkali content of the fly ash. ASTM C618 has no limit on alkali content. Because fly ashes are so different from one another, you need to test the effectiveness of each material combination. ASTM C1778 provides a protocol for evaluating aggregate susceptibility and potential control measures. Restricting the alkali content or prohibiting Class C fly ash won’t address the problem; only testing will do.
Some specifications limit the carbon content (loss on ignition) of fly ash. This restriction is aimed at the effect on air entrainment, as carbon adsorbs surfactant admixtures. However, if the fly ash is consistent, it shouldn’t be too difficult to control the air content. It may be necessary to adjust the dosages of the air-entraining and water-reducing admixtures. But the contractor should be able to figure out whether the cost of the additional admixtures is worth the ability to use the available fly ash.
Getting from prescription to performance
[S]pecifications should capitalize on the expertise of the concrete producer and the contractor—in the former case, for development of concrete mixtures, and in the latter case, for construction means and methods. Prescriptive specifications that describe the details of concrete mixture parameters are constraints against achieving these objectives. With prescriptive specifications…the concrete producer is often held responsible if there is any problem with concrete on a project. This violates a basic principle that responsibility and authority should be congruent.—Obla and Lobo
Engineers need to understand what performance is necessary, how tests correlate with performance, and how to test for quality assurance. Many engineers aren’t familiar with test methods. When I worked for a testing lab, I sometimes had to advise engineers that they needed to specify not only the test, but also the required result.
Most people copy and paste rather than write specifications from scratch. However, test methods and building codes receive periodic updates. Specifications should incorporate the most current versions.
A colleague once asked me how to price a Michigan DOT test for a job he was bidding. He’d looked up MDOT’s specification but couldn’t find the test. I called an acquaintance at MDOT, who found the relevant pages in an old file. The test determined whether fresh concrete contained corrosion inhibitor. It involved weighing a sample of fresh concrete, mixing it with water, letting it settle, and collecting a sample of the supernatant liquid to analyze in the laboratory. Of course we’d have to do all this on a construction site, and the laboratory analysis would likely take several days. MDOT no longer used that test because a simple test strip could give the same result in seconds—right there on the job site. Yet the old test lived on in specifications.
Certification and enforcement
Another concern is ensuring that the testing agency can conduct the tests accurately and consistently. As we’ve discussed previously, improper testing of compressive strength increases the producer’s risk of rejection of good concrete. Various organizations accredit laboratories such as Beton’s and certify technicians to ensure the minimum qualifications. But that’s not enough. Studies showing that a majority of projects have improper testing involved certified technicians. How can we ensure that the technicians do the job they know how to do?
And what about tests other than compressive strength? To conduct any test consistently, technicians need to stay in practice. For less common tests, even a large lab may have only one or two technicians with the necessary qualifications and experience.
One important consideration is that proper testing is not cheap. Maintaining the certifications, calibrations, records, and oversight costs money. There’s a lot of internal quality work that the client may never see, but in the long run is evident in the reliability of the testing. Because cheap testing can be expensive in the long run, it’s imperative not to award testing contracts on the basis of the low bid. Low-bid contracts act as perverse incentives, encouraging testing labs to cut corners instead of doing meticulous work. The specification should also spell out who is responsible for providing on-site curing and what it requires so there’s adequate payment for it. ACI PRC 132.1 spells out the responsibilities of the various parties in more detail. It’s a good example of “think first, then do.”
Ultimately, both laboratories in particular and the construction industry in general need to foster a culture of good testing. It may cost more up front, but it saves money in the long run. And as the industry moves from prescription to performance, reliable testing is essential.