Climate change is already affecting everything from wildfires to coral reefs. To combat climate change, the cement industry has announced its 2050 Carbon Ambition, pledging to produce carbon-neutral concrete by 2050. What would it mean to have net zero concrete, and how do they propose to go about it?
We can produce net zero concrete buildings today by following the practices of CALGreen. Combining concrete’s insulating characteristics and heat capacity to maintain comfortable interior temperatures with an energy-efficient HVAC system goes a long way toward carbon neutrality. Where it’s practical, deep water cooling can dramatically reduce the carbon footprint for air conditioning. You can go even further by mimicking termite “architecture” to promote convection currents inside the building. Beyond that, minimizing and reusing waste materials, installing solar panels, and designing for durability all contribute to the end result. That is, over the lifetime of the building, it can be carbon neutral.
Structural design for a lower carbon footprint
Structural design can make more efficient use of concrete to reduce the carbon footprint. Using less concrete means using less cement.
There are different ways to do this. One is to use high-strength concrete for smaller cross sections. For example, in a high rise building, the columns on the lower floors have to be much larger in 6000 psi concrete than in 12,000 psi concrete. Concrete that’s twice as strong doesn’t need twice as much cement, so high-strength concrete could save on cement. As a bonus, smaller columns mean more usable floor space.
The engineer could also use high-strength concrete in floor slabs to permit longer spans, which means that fewer girders and columns can support the same floor area. More open space in the interior also makes for more flexibility for the floor plan.
Similarly in a bridge, fewer girders and piers could support the same bridge deck. Reducing the number of bridge piers can result in other benefits, such as less interference with river traffic or aquatic wildlife.
Prestressed concrete
The engineer could also use prestressing or posttensioning. These two methods are the same in principle, but the execution is different.
In prestressing, steel tendons are stressed before the concrete is placed. The concrete consolidates around them, sets, and gains strength. With steam curing, the forms and the external tension are removed in 16 to 18 hours. The tendons start to go back to their original length, but the concrete restrains them. That leaves tension in the steel and compression in the concrete before it takes on any external load. Because concrete is much stronger in compression than in tension, this is a highly efficient use of the material. That is, each material plays to its strengths.
In posttensioning, ducts are cast into the concrete. After the concrete has reached the required strength, steel tendons are threaded through the ducts, tensioned, and anchored at the ends. The ducts may be filled with grout.
Portland cement manufacture
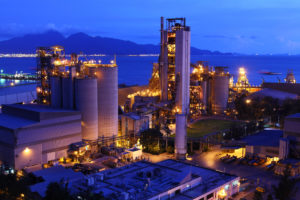
Traditionally, we made concrete from portland cement, sand, coarse aggregate, and water. Nowadays at least one supplementary cementitious material usually displaces some of the portland cement. And we usually use chemical admixtures as well. (Judicious use of chemical admixtures can reduce the portland-cement content as well.) But generally portland cement is still a major component. And that’s a problem if you want to reduce concrete’s carbon footprint.
To see why, you need to know something about how we make portland cement. It starts with extracting the component materials from a quarry. These may include limestone, clay, shale, sand, and iron ore. Anything that’s in large pieces must be crushed to a usable size before further processing. This process, which we call comminution, is energy intensive and often inefficient.
Then we combine the component materials in the correct proportions and feed them into the cement kiln, which heats them to a clinkering temperature of about 1500 ˚C. Naturally, that burns a lot of fuel. But that’s only part of the carbon footprint. During clinkering, the limestone (CaCO3) breaks down, giving off CO2 gas.
The clinker particles need to cool before grinding. During grinding, small amounts of gypsum and sometimes other ingredients are added. Like the comminution of the kiln feed components, grinding clinker is energy intensive and inefficient.
Traditionally, producing one ton of portland cement also produced one ton of CO2 emissions.
Making portland cement clinker less energy intensive
Cement manufacturers have worked for decades to reduce the embodied energy in portland cement. From the beginning, they located their plants near the quarries to avoid having to transport raw materials.
Naturally they have concentrated on saving fuel in clinkering. Originally, they mixed the raw kiln feed with water to make a slurry. However, evaporating that water makes for a highly inefficient process, so modern cement plants use a dry process. They add fluxes to reduce the clinkering temperature. They also use waste heat from the kiln to preheat the raw kiln feed.
Some cement plants use waste materials as fuel or as part of the raw kiln feed. This can be a highly efficient practice, as it saves on having to quarry and crush raw materials. One plant burns scrap tires in its kiln, using the rubber as fuel and the steel belting as an iron source for the cement. It also burns waste solvents, inserting them into the hottest part of the kiln so that the toxic molecules completely dissociate into harmless components. Some plants are beginning to recycle wind turbine blades as kiln feed.
Other plants incorporate high-carbon fly ash into their raw kiln feed. This fly ash isn’t suitable for use directly in concrete because the carbon interacts with some admixtures, making them less effective. But in the kiln the carbon becomes fuel and the other components of the fly ash displace virgin materials that would require quarrying and crushing before use. The fly ash is already so fine that it needs no further comminution.
More efficient comminution
Because comminution requires a lot of electrical energy, manufacturers have looked for ways to make it more efficient as well. Adding grinding aids to the mill is a longtime practice. However, it is even more useful to remove material from the mill as soon as it’s fine enough. Otherwise the fines just cushion the impact of the grinding—that is, they get in the way of further grinding—and may be ground too finely themselves.
Beginning in the 1980s, cement manufacturers started installing high efficiency separators in their mill circuits. The separator subjects the cement particles to a cyclonic air current, flinging them to the walls. The large, heavy particles slide down the walls and out the bottom, while the smaller, lighter particles remain suspended. That way the large particles go back to the mill for further grinding, while the fine particles go into the silo to cool instead of interfering with the grinding.
Towards net zero concrete
Despite all these advances in energy efficiency, however, there’s no getting away from the production of CO2 gas due to the breakdown of the limestone in the kiln. That is, so long as there’s portland cement in it, it won’t be net zero concrete. Some cement manufacturers offer carbon offsets to compensate for this.
Cement manufacturers have worked to reduce the amount of portland cement clinker in their products. They produce various kinds of blended cements, which contain fly ash, calcined clay, silica fume, and/or slag cement along with the portland cement. Accurate acceptance testing allows concrete producers to minimize the amount of cement they need to achieve the design strength.
Of course, one could also produce similar combinations at the ready-mixed concrete plant. However, blended cements afford certain advantages. The cement producer can adjust the gypsum content to control the setting of that specific combination of materials, while the concrete producer doesn’t have that option. Also, silica fume can be hard to mix thoroughly in the ready-mix plant, but is fully blended in blended cement. In addition, a blended cement needs only one silo at the ready-mix plant. And concrete producers can always add supplementary cementitious materials to a blended cement if they want to.
Another way cement manufacturers reduce the amount of clinker in their cements is with interground limestone. That is, they add raw limestone into the cement mill so that the clinker and limestone grind together. The limestone itself does not hydrate, but its fine particles help to accelerate the hydration reactions of the portland cement clinker. Being softer than clinker, the limestone grinds finer.
2050 Carbon Ambition
As mentioned above, the cement industry has pledged to make net zero concrete by 2050. This is their six-point strategy.
- Eliminate energy-related emissions and make more use of waste fuels from other industries. Examples of the latter include tires, waste solvents, and high-carbon fly ash.
- Use renewable energy sources where available. For comminution, the electricity could come from solar or wind generation.
- Reducing process emissions and employing carbon capture. Because CO2 gas comes from limestone as well as burning fuels, carbon capture is essential so long as portland cement clinker is part of the product. However, for now the technology is not ready to implement in cement manufacture.
- Reducing the amount of clinker in cement and cement in concrete, as well as more efficient use of concrete. We’ve already discussed supplementary cementitious materials in blended cement and concrete. Another way to use less cement is to proportion the concrete using the aggregate suspension method, which maximizes the aggregate content.
- Recycling concrete from construction and demolition waste for use as aggregates. One way to do this is to reuse fresh concrete.
- Enhancing recarbonation of concrete. Dave Borlace discusses some of the relevant technologies in his “Just Have a Think” video.
Geopolymer cements
2050 is a long way off. What can we do now to achieve net zero concrete? One possibility is to eliminate the portland cement altogether using geopolymers. These are combinations of supplementary cementitious materials and sometimes other waste materials that together can perform the function of cement in concrete. Today, the vast majority of concrete contains at least some portland cement because these other materials tend to react much more slowly. That means it takes much longer for the concrete to gain the necessary strength and other properties. If we don’t want to wait, we can add chemical activators or heat the concrete to speed things along. However, the chemicals can be hazardous, and of course heating takes energy and usually emits CO2.
An alternative approach is simply to accept that geopolymer concrete takes longer to set and gain strength and plan accordingly. Contractors do this already when using fly ash or slag cement. They schedule their finishers to come in later because they know they need to wait until the concrete is ready to finish. A contractor who knows how much longer the concrete will take to set and gain strength can plan the construction sequencing so the slow reaction times don’t delay the project. Maturity testing in the laboratory while we’re verifying the concrete mixture can inform these decisions.
We are starting to see specifications that set limits on the embodied carbon in the concrete. Beton is a leader in developing and using alternative cementitious materials. We know how to make them work in practice, not just in the laboratory, and we can help you meet this kind of specification.